Making Radio Galaxies in a Computer
At a conceptual level the formation of radio galaxies is pretty simple. According to a basic picture first introduced in the 1970s, a supermassive black hole in the center of a galaxy generates a symmetric pair of oppositely directed, high speed jets or beams of hot, ionized gas as a by-product of energy released or stored from matter falling onto the black hole. Those jets drill holes in the atmosphere of the galaxy and then even far beyond, dumping energy, excavating cavities and possibly entraining gas into the jets and cavities along the way. The jets carry magnetic fields and high energy electrons. Those electrons, spiraling in the magnetic fields light up the jets and the cavities they excavate in the radio band through a process called synchrotron emission.
While calculations based on this cartoon picture can correctly predict a few properties of radio galaxies, anyone who has looked at the images in Radio Galaxy Zoo can see that there must be a whole lot more to the story. Radio galaxies at best have only a rough bilateral symmetry with respect to their host galaxies. Furthermore, no two radio galaxies look alike, and most look pretty complicated; some could only be described as messy. In fact, the physics of radio galaxy formation is really very complex for a whole bunch of reasons that range from inherent instabilities in the dynamics of a fast jet, to the reality that the jets are not steady at the source. Furthermore, the surrounding environments are themselves messy, dynamic and sometimes even violent. All of these influences have impact on the appearances of radio galaxies.
The other side of the coin is that, if they can be understood, these complications may improve opportunities to decipher both the formation processes of the jets as well as the conditions that control their development and dissipation as they penetrate their environments. One part of piecing this puzzle together is expanding our awareness of all the things radio galaxies do, as well as when and where they do what they do. That’s what Radio Galaxy Zoo is about.
On the other hand, to go beyond the cartoon picture of what we see we also have to develop much more sophisticated and realistic models of the phenomena. This is very challenging. Because the detailed physics is so complex (messy!), astronomers have come to depend increasingly on large computer simulations that solve equations for gas dynamics with magnetic fields and high energy electrons. Pioneering gas dynamical simulations of jets in the 1980s already played an important role in confirming the value of the jet paradigm and helped to refine it soon after it was introduced.
Those early simulations were, however, seriously limited by available computer power and computational methods. In important ways the structures they made did not really look much like actual radio galaxies. At best they were too grainy. At worst important physics had to be left out, including the processes that actually produce the radio emission. This made it hard to know exactly how to compare the simulations with real radio galaxies. Thankfully, rapid improvements in both of those areas have led recently to much more realistic and detailed simulations that are starting to look more like the real thing and can be used to better pin down what is actually going on.
Our group at the University of Minnesota has been involved for some years now in pushing forward the boundaries of what can be learned about radio galaxies from simulations. I illustrate below some of the lessons we have learned from these simulations and some of the complex radio galaxy environments that it is now possible to explore through simulations. Each of these simulations was part of the work carried out by a student as part of their PhD training.
The jets responsible for radio galaxy formation propagate at speeds that can be a significant fraction of the speed of light. They are almost certainly supersonic. These properties lead to several related behaviors that are illustrated in Figure 1. It turns out that the flows within such a jet tend periodically to expand and then to contract. As they do so they form a sequence of shocks along the jet. These are visible in the figure. The jet also creates a sonic boom or bow shock in front as it moves forward. A close look at the jet in this figure also reveals that the jet actually does not remain straight as it moves forward. The end of the jet turns out to be unstable, so soon after launch begins to ‘flap’ or wobble. As a result the end of the jet tends to jump around, enlarging the area of impact on the ambient medium.

Figure 1: Shock waves in a simulated high speed jet propagating from the left end of the box. Since the jet is driving supersonically through its ambient medium, it also has created a bow shock (sonic boom) in the ambient medium on the far right.
Many radio galaxies form inside clusters of galaxies, where the ambient medium is highly non-uniform and stirred up as a result of its own, violent formation. This distorts and bends the radio structures. At the same time the energy and momentum deposited by the jets creates cavities in the cluster gas that lead to dark holes in the thermal X-ray emission of the cluster. Figure 2 illustrates some of these behaviors for a simulated radio galaxy formed at the center of a cluster. Even though the source of the radio galaxy is at rest, there are fast gas motions in the cluster gas that obviously deflect the radio galaxy jets. ‘Mock’ radio images representing synchrotron emission by high energy electrons in the magnetic field carried by the jets are shown on the right in the figure at two times. At the same two times mock images of thermal X-rays are shown to the left. The X-ray images have been processed to exaggerate the dark cavities produced by the jets. Note that each image spans about 700 kpc or 2 million light years.
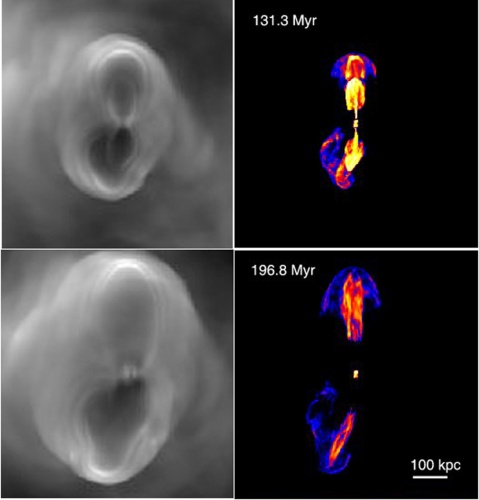
Figure 2: Simulation of radio galaxy formation in a cluster of galaxies that was extracted from a separate simulation of galaxy cluster formation. Mock observations of thermal X-rays (left) and radio synchrotron emission (right) are shown at two times after a pair of oppositely directed jets formed in the cluster center.
Quite a few radio galaxies in clusters are not made by galaxies anchored in the cluster center, but are hosted by galaxies moving through the cluster. This is especially common in clusters that are in the process of merging with another cluster. In that case the host galaxy can be moving very fast, and even supersonically with respect to its local, ambient medium. Then the radio jets can be very strongly deflected into ‘tails’ by an effective cross wind and eventually disrupted. Figure 3 illustrates the mock synchrotron emission from such a simulated radio galaxy. The abruptness of jet bending depends on the relative speed of the jet with respect to its internal sound speed and the relative speed of the host galaxy through its ambient medium with respect to the sound speed of that medium. So, when strongly bent jets are seen in a radio galaxy it is a strong clue that the motion of the galaxy is supersonic in relation to its environment. When multiple tailed radio galaxies are found in a given cluster it provides potentially valuable information about the dynamical condition of the cluster, since a relaxed cluster ought not to have many galaxies moving at supersonic speeds through the cluster gas.

Figure 3: Mock synchrotron image of a simulated radio galaxy embedded in a supersonic cross wind. The two jets have bent into downstream, turbulent tails .
Even more complex motions between the host galaxies and the ambient gas are possible. Those can sometimes lead to really exotic-looking radio structures. One beautiful example of this is the radio source 3C75 in the merging cluster Abell 400. Evidently two massive galaxies have become gravitationally bound into a binary system with a separation of about 7 kpc. The orbital period should be around 100 Myr. The pair also appears to be moving together supersonically through the ambient medium. Each of those galaxies has formed radio jets. If it were not for the binary the expected outcome might resemble the situation pictured in Figure 3. However, the binary motions cause each of the two galaxies to oscillate in its motion and this causes the radio jets to develop more complex, twisted shapes before they disrupt into tails. Figure 4 illustrates a preliminary effort to simulate this dynamics. The image on the right shows the real 3C75, where pink is the radio emission (VLA) and blue is thermal X-rays (Chandra). The image on the left traces the distribution of gas expelled by each of the two galaxies in the binary system. This simulation seems to capture the general character of the dynamical situation responsible for 3C75.

Figure 4: Right-the radio galaxy 3C75 as seen in the radio (pink) and associated thermal X-rays from the cluster Abell 400 (blue). Left-Simulated jets from a binary pair of galaxies intended to mimic the dynamics of 3c75.
From this short set of simulation results it ought to be clear why many different kinds of radio galaxy structures are expected to form. It also ought to be apparent that we need better catalogs of what behaviors do exist in nature in order to see how to focus our simulation efforts and to establish what are the most important dynamical conditions in radio galaxy formation.
I find it amazing that events at such tiny scales as SMBH and its immediate environs (no bigger than ~the size of the solar system) can produce such gigantic structures as Mpc-wide jets and lobes! How do you successfully simulate physics which covers such a huge range of scales?
A question: in the first para “The jets carry magnetic fields and high energy electrons” Surely the jets also carry at least protons (hydrogen nuclei) and alpha particles (helium-4 nuclei), and possibly highly ionized ‘metals’ too? And while ‘carry’ isn’t the right verb, the jets are also beams of very high energy neutrinos, aren’t they?
Thanks for your interest. First, it is indeed very difficult to simulate the full range of scales from the size of the solar system to the size of a cluster of galaxies in a single simulation. Impossible, actually. So, instead, simulations generally focus on either the source end of the scales (how do you make a jet realistically) or what happens to a jet once it is made. The simulations I show in the blog are of the latter type. They follow events only after the scales get to kpc and bigger. We utilize the results of other simulations that zoom down to SMBH scales in order to create our jets.
As to the composition of jets, that is still an unanswered and debated question. It probably depends actually on where you are. It is quite possible, and some simulations suggest, that at the source near the SMBH the jets consist almost entirely of electromagnetic energy along with electron/positron pairs that are products of that electromagnetic energy. Some models do begin with substantial proton loads. Then, either if there are also neutrons or the protons collide with other protons, the jets, as you suggest,would also generate a neutrino flux. The neutrinos would not generally follow the dynamics of the other components, however, once they were generated.The biggest worry about models with big ‘baryon loads’ is that if the proton load is high then the total energy requirements for the jets become very large. The minimum energy requirements for the most powerful jets are already hard to explain even if the ‘baryon load’ is small. Independent of their composition at the beginning, jets will eventually entrain ordinary matter, which, as you suggest would contain all the usual ingredients. But, again it is hard to explain the most powerful jets if this entrained gas is well mixed in. Furthermore, measurements of the so-called Faraday rotation effect in polarized radio emission puts pretty tight limits on the amount of this gas in at least the most powerful jets. It is possible and often suggested that many jets may contain fast cores devoid of anything but electromagnetic energies and electron/positron pairs, although that is not established. Some jets, and probably the most severely distorted jets are likely to contain significant amounts of entrained gas. Our simulations are intended partly to study this process, actually.
In case the question comes up, it turns out that the annihilation rates of any electron/positron populations in the jets are too small to lead to detectable gamma rays from that process. Other sources of gamma rays will overwhelm that.
As to terminology, the verb ‘carry’ is correct, I think, since it reflects what you find if you put yourself in the local rest frame of the jet plasma, whatever it is. So long as there is any matter of any kind in the jet, this is something you can do. The terms ‘jet’ and ‘beam’ get used interchangeably in this community. They represent different aspects of what is going on, just as you can call a stream of water coming from a hose a jet or a beam. The former term reflects the fact that it moves fast, but has internal ‘thermodynamics’, while the latter reflects the fact that it transports energy and momentum at high speed. Both are valid, but incomplete.
Thanks for the information-packed and lengthy reply! 🙂
I have lots, and I mean lots, of questions; perhaps I should ask on RGZ Talk?
Anyway, here are just a couple … at the source near the SMBH the jets consist almost entirely of electromagnetic energy along with electron/positron pairs that are products of that electromagnetic energy. Yeah, this is about something other than your simulations, but still … “electromagnetic energy”? What is this? Intense electric and magnetic fields, generated by the residual charge of the SMBH? UHE gammas trapped near the event horizon/in the ergosphere?
If the jets have only a small baryon load (lovely term!), and comprise predominantly electrons, what prevents them from being braked (probably not the best verb) by whatever is the relativistic equivalent of a Debye sphere?
All right, three questions … when you ‘look down the barrel’ of your simulations, do you ‘see’ things which resemble BL Lac objects, SEDs, polarizations, variability and all?